3D Echocardiography
3D echocardiography in dogs and cats
The first 3D echocardiographic images saw the daylight almost 50 years ago. When Dekker et al rendered several 2D images into a 3D structure (1). Approximately 20 years later, real-time 3D, sometimes called 4D due to the live feed, was developed by Von Ramm et al (2,3). Since then the technology has developed with new generations of transducers: the fully sampled matrix array transducers. These transducers do not only transmit sound in two planes but also laterally in multiple directions. This lateral transmission is made possible by multiple rows and columns of piezoelectric elements in the transducer. Together, the voxels or volumetric pixels build up a pyramidical volume. Currently, the transducers contain up to > 6400 elements, a vast increase from the first generation of (sparse) matrix array with 156 elements (4).
Despite the technological improvement, 3D echocardiography is still considered by many to be more of a lab gadget than a clinical tool.
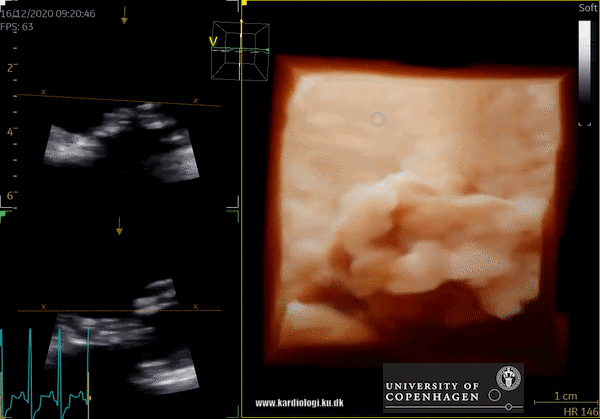
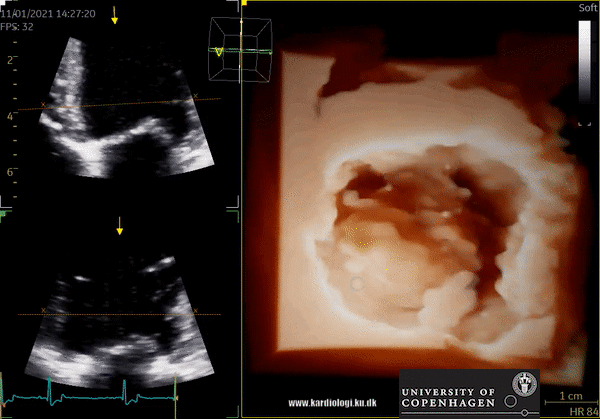
3D Data acquisition
Voxel-based 3D echocardiography can acquire data by two different methods; 1. ECG-gated 3D or 2. Real-time (live) 3D echocardiography.
1) ECG-gated 3D echocardiography is a mode where several heartbeats and partial volumes are recorded and stitched together to create one volume. This is sometimes called near real-time 3D, as it is not strictly real-time. The ECG-gated mode makes it possible to generate large volumes with high spatial and temporal resolution, but often at the cost of stitch artefacts. Stitch artefacts are common artefacts where the volumes are not stitched together optimally. This is most often caused by movement such as respiration and arrhythmias. Naturally, more recorded heartbeats increase the possibility of stitch artefacts.
2) In real-time 3D echocardiography, the volume is obtained during one single heartbeat. This mode does not create stitch artefacts since it’s derived from one heartbeat. The greatest trade-off with real-time 3D echocardiography is the relation between spatial and temporal resolution. A way to enhance spatial resolution while not giving up frame rate is to narrow down the observed volume (5,6).
Another possible image acquisition with the matrix array transducer is the 3D-guided multiplane mode, which can be further categorized in biplane and triplane mode. While this mode doesn’t capture a volume but several orthogonal 2D images, multiplane has proven to be beneficial. Multiplane has similarities to conventional 2DE but with one major difference; it utilizes the possibility of 3D to view the LV from different perspectives, and thus enabling the possibility of obtaining the true long and short axis of the LV. This is accomplished by first manually finding the position of LV apex in one of the views. Afterwards, the software can automatically position the apex in the other views (7). After manually delining the endocardial border in both EDV and ESV the LV volume can be calculated similarly to the biplane discs method. Like 2DE, this is still a geometrical assumption, but often a more accurate assumption (7,8).
For assessment of the LV, a data acquisition set from the apical view is recommended (9).
Assessment of the LV volume with voxel-based 3DE
Both on- and off-line analysis tools can extract LV volume from the obtained data. In general, a semi-automatic tracing program follows the endocardial border by tracking speckles or greyscale differences between the endocardium and adjacent tissue around the entire LV. Initially, however, the analyst needs to inform the software where certain landmarks are located in EDV and ESV. The landmarks used for the LV are the mitral annulus and LV apex. The obtained volume can then be examined and manually retraced if the software fails to follow the endocardial border (8,10).
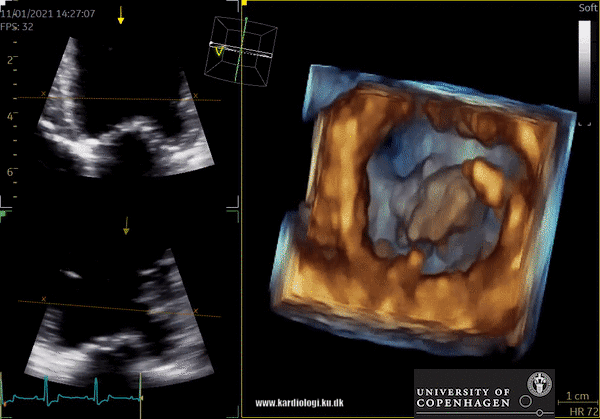
Display of voxel-based 3D data - crop till you drop😊
Once a volume has been obtained the analyst can post-process the volume. This is unique in the sense that it allows the operator to crop away unwanted volume and information. Additionally, the operator can, much alike conventional echocardiography, change image settings such as gain and brightness (10). However, there’s a limit to the amount of gain and compression that can be added or removed in post-processing, so the best possible settings during examination are recommended (10).
After post-processing, the volumetric data can be displayed. Currently, a general agreement on how to display 3D images does not exist, although different visualization techniques are recommended for different clinical structures and purposes (9,11). The techniques that are used to visualize the obtained data are:
1) 2D Tomographic Slices.
In short, this view allows the operator to view the chamber with either multiple slices or orthogonal planes. In theory, this makes it possible to view the chamber from any cutting plane. In reality, poor spatial-temporal resolution can prevent such acquisition (9,10).
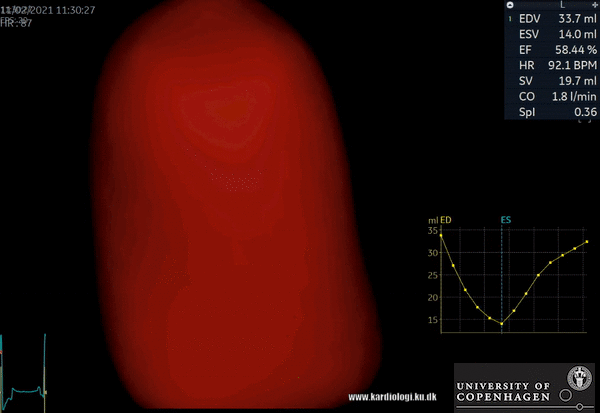
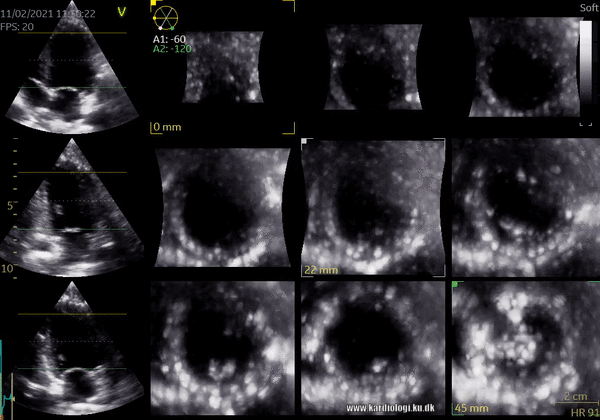
2) Surface rendering:
In this view, the surface of the chamber can be evaluated. It can be seen either as a solid or a wireframe volume. Further stereoscopic viewing can help in visual assessment of the shape (9).
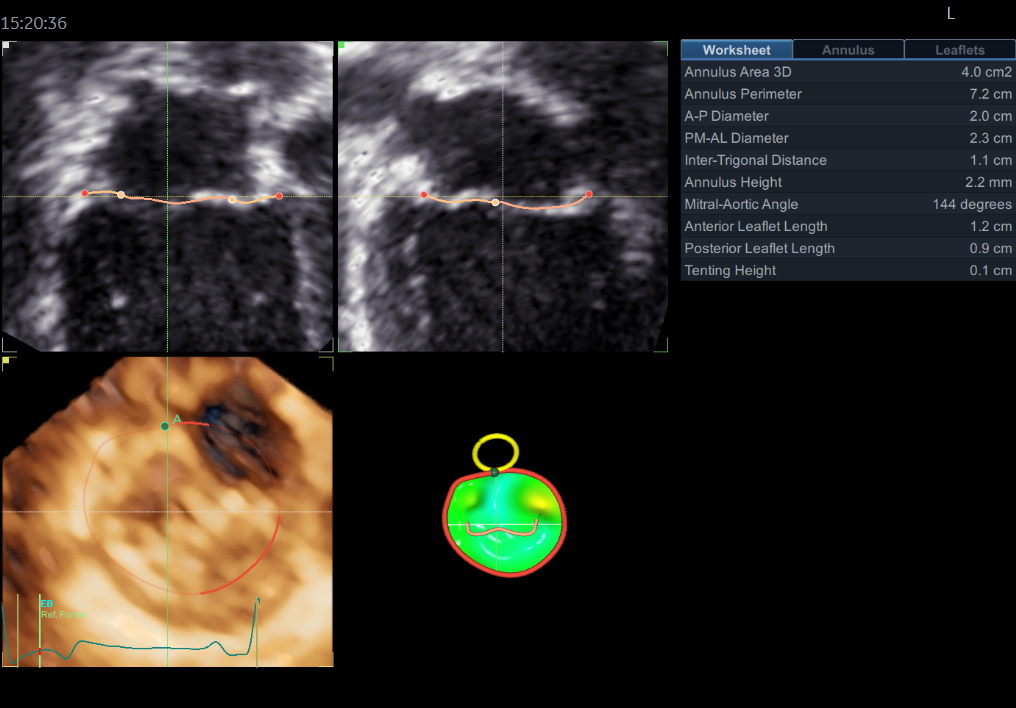
3) Volume rendering:
This visualization technique gives a view through the cropped volume and hence a depth perception. This can be useful in the evaluation of for example the mitral annulus (9).
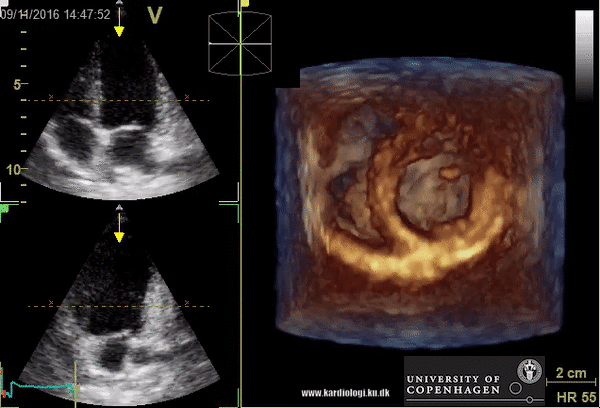
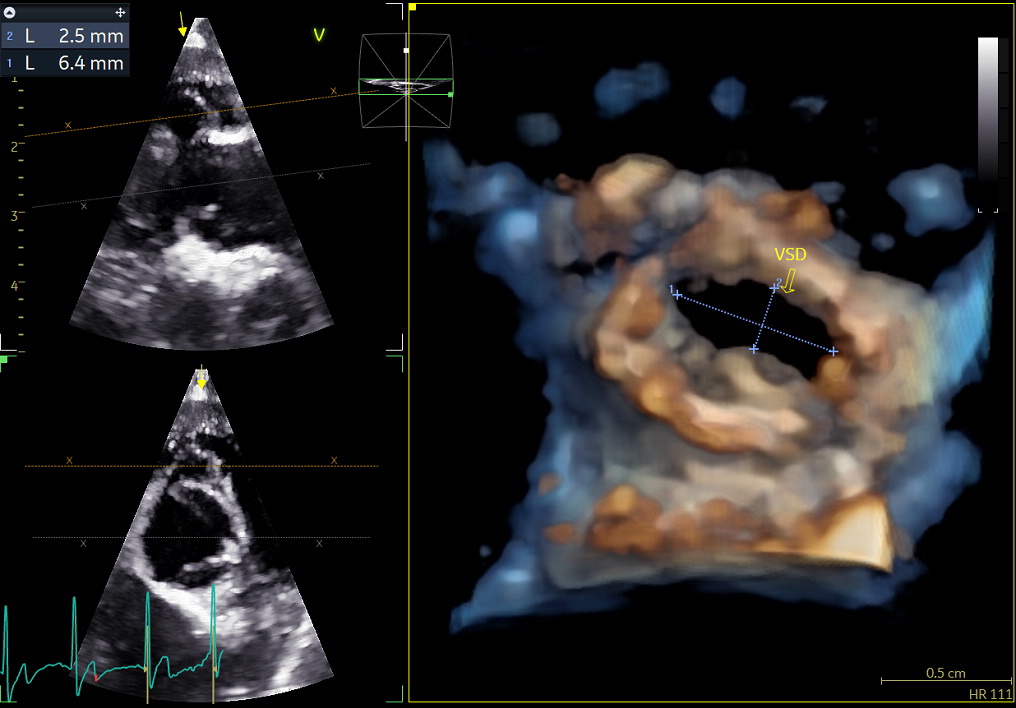
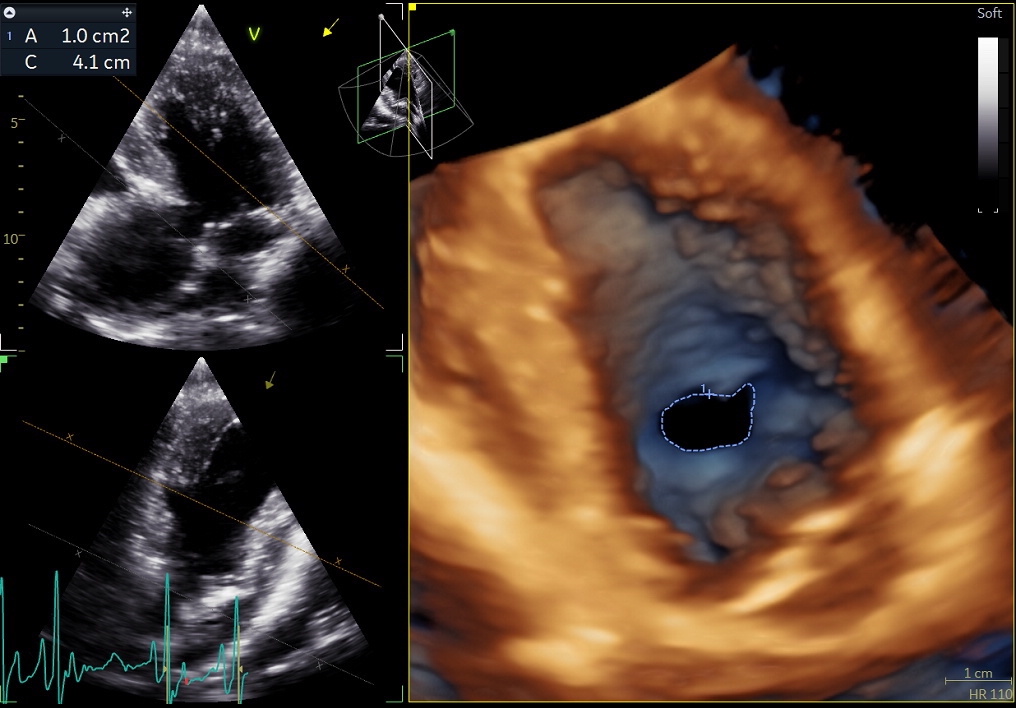
Benefits of 3D echocardiography in LV assessment
Accuracy
Studies show that the volume obtained through 3D echocardiography correlates to a higher degree with the golden standard, cardiac MRI, than that of M-mode and MOD BP. Additionally, studies in 3D echocardiography also proved to correlate to a higher degree with cardiac MRI than CT, although with higher variability.
Reproducibility
Studies also demonstrated that 3D echocardiography has a higher reproducibility than M-mode and MOD BP, thus demonstrating 3D echocardiography to be less operator-dependent than these conventional modalities.
Feasibility
While off-line analysis is not considered a clinically practical approach. On-line analysis is possible and considered feasible in a study by Jenkins et al (12). Even though the on-line approach yielded accuracy significantly less accurate than the off-line analysis, it still produced significantly more accurate results than 2D while being less time consuming than the off-line approach.
Multiplane Echocardiography
With 3D-guided multiplane echocardiography, the acquisition time is reduced while not giving up significantly on spatial and temporal resolution, compared to conventional 2D images. This is due to the ability of multiplane to simultaneously capture images without the need to rotate the probe. As previously mentioned, this reduces the possibility of errors in LV volume measurements due to foreshortening. Several studies have even reported 3D guided triplane echocardiography to be superior in accuracy than conventional and voxel-based 3DE, yielding results not significantly different from cardiac MRI. These studies conclude that triplane echocardiography is rapid and easy to perform. Multiple studies have also demonstrated higher reproducibility with triplane echocardiography than conventional 2DE. Furthermore, in a study by Nucifora et al, the overall measurement times to display EF were (mean ± SD) 241 ± 49 seconds for 2DE and 175 ± 54 seconds for triplane echocardiography (7).
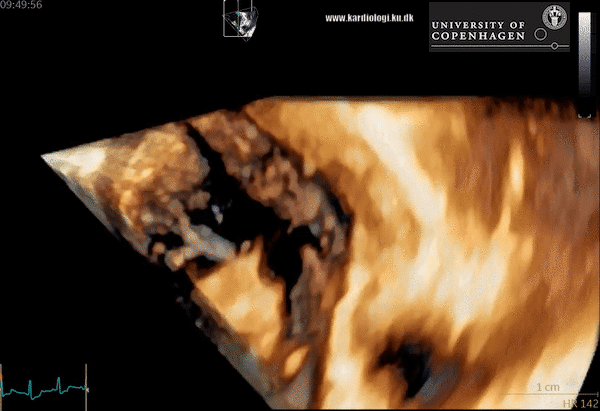
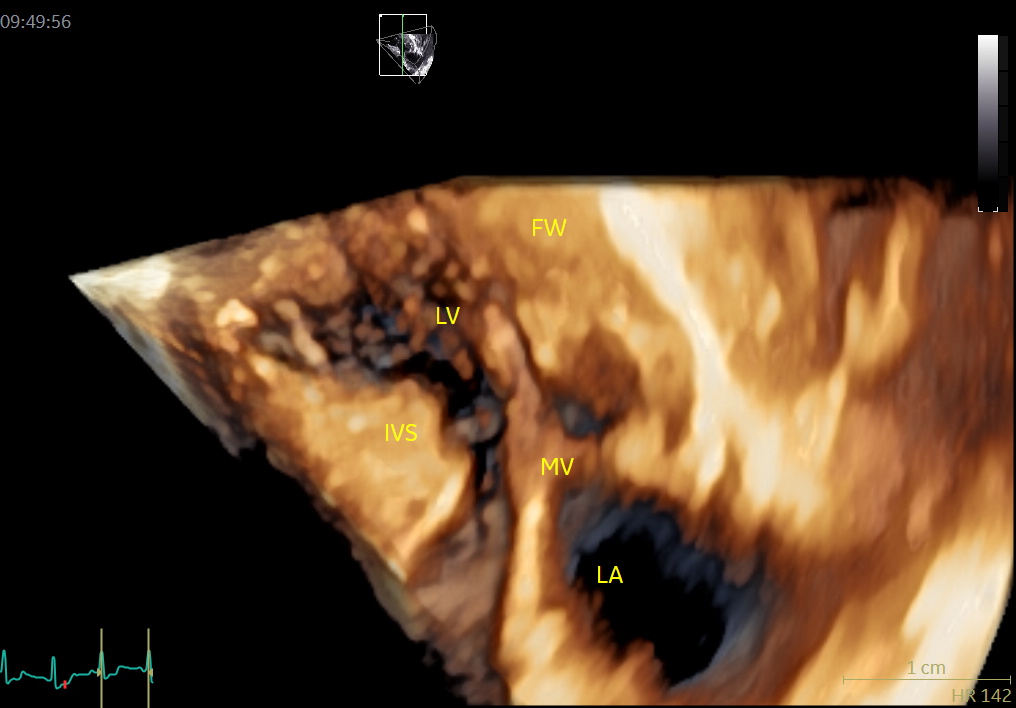
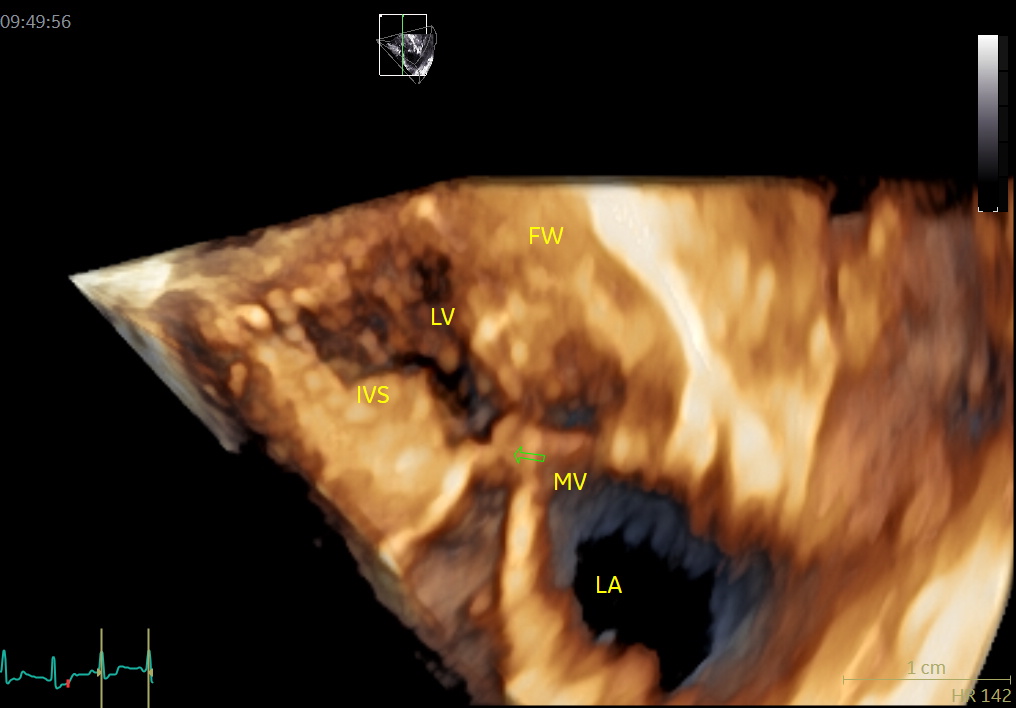
An image with an obstructed LVOT (arrow) in late systole. MV = mitral valve, IVS = basal septal hypertrophy, FW = LV posterior wall, LV = left ventricle, LA = left atrium.
Limitations of 3D echocardiography
Boundary tracing
Voxel-based 3DE is not dependent on geometrical assumptions, but it’s highly dependent on the software to locate the endocardial border exactly. Research has shown that even slight differences in the scale of millimeters when tracing the endocardial border can result in great volumetric errors. In a study by Muraru et al voxel-based 3DE were often in need of manual adjustment for greater accuracy (13).
Spatial-temporal resolution
Voxel-based 3DE poor spatial-temporal resolution is considered the cause of reported volume underestimation. With data of poor resolution, the 3D software has problem distinguishing of the endocardial trabeculae from the myocardium, which leads to an underestimation, as the trabeculae should be included in the LV volume.
The highest spatiotemporal resolution is obtained from an ECG gated acquisition. However, patients with arrhythmias or patients unable to hold their breaths are challenging with ECG gated acquisition, as they are likely to obtain stitching artefacts during the acquisition.
Time consumption
Voxel-based 3DE requires less time to capture the volume of interest. This is especially useful during stress echocardiography where a short acquisition time is advantageous. However, the analyzing time after the acquisition is longer, both on- and off-line. When the manual correction is needed for border tracing, analyzing time is increased.
Computer storage
At the moment a combined voxel-based 3DE and 2DE exam require on average approximately 5-10 times as much digital space than a 2DE exam alone. Lang et al note that the large data sets can overwhelm the system with regards to the transmission and storage process (9).
Probe size
Due to increased complexity, the transducers have grown in size from the conventional 2D probes. We have two 3D probes (ranging from a <2 kg to > 80 kg dog). Small probe footprint is necessary to gain access through the intercostal space in small animals.
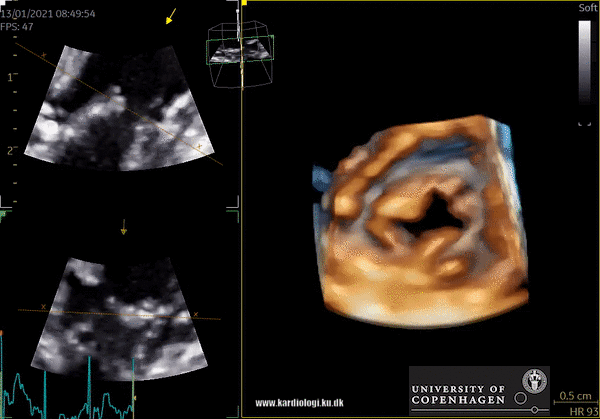
Cost
Currently, high cost may prohibits a widespread veterinary clinical use.
Authors:
Sven Sundin, DVM, Jakob Willesen, DVM, PhD, Jørgen Koch, DVM, PhD
References
- Dekker DL, Piziali RL, Dong E.: A system for ultrasonoically imaging the human heart in three dimensions. Comput Biomed Res. 1974; 7:544-53.
- Sheikh KH, Smith SW, Von Ramm O, Kisslo J.: Real-time, Three-dimensional echocardiography: Feasibility and initial US. Echocardiography (Internet). Available from: https://doi.org/10.1111/j.119-125.75.1991.tb01409. x.abstract
- Badano LP, DallÀrmellina E, Monaghan MJ, Pepi M, Baldassi M, Cinello M, et al.: Real-time three-dimensional echocardiography: technological gadget or clinical tool? J Cardiovasc Med (Hagerstown). 2007;8:144-162.
- Nanda NC, Hsiung MC, Miller AP, Hage FG.: Live/real time 3D echocardiography chapter 3: How to do a 3D echocardiogram: examination protocol and normal anatomy. Blackwell Publishing Ltd; 2010. 23-54.
- Macron L, Lim P, Bensaid A, Nahum J, Dussault C, Mitchell-Heggs L, et al.: Single-beat versus multibeat real-time 3D echocardiography for assessing left ventricular volume and ejection fraction: A comparison study with cardiac magnetic resonance. Circ cardiovasc Imaging. 2010; 3:450-55.
- Chang SA, Lee SC, Kim EY, Hahm SH, Jang SY, Park SJ, et al.: Feasibility of single-beat full-volume capture real-time three-dimensional echocardiography and autocontouring for quantification of left ventricular volume: Validation with cardiac magnetic resonance imaging. J Am Soc Echocardiogr (Internet). Elsevier Inc; 2011; 24(8):853-59. Available from: http:/dx.doi.org/10.1016/j.echo.2011.04.015
- Nucifora G, Badano LP, Dall´Armellina E, Gianfagna P. Alocca G, Fioretti PM.: Fast data acquisition and analysis with real time triplane echocardiography for the assessment of left ventricular size and function: A validation study. Echocardiography. 2009; 26(1):66-75.
- Mor-Avi V, Lang RM.: Echocardiography Quantification of left ventricular volume: What can we do better? J Am Soc Echocardiogr. 2008;21:998-1000.
- Lang RM, Badano LP, Tsang W, Adams DH, Agricola E, Buck T, et all.: EAE/ASE: Recommendations for image acquisition and display using three-dimensional Eur Heart J Cardiovasc Imaging (Internet). 2012 Jan, 13(1):1-46. doi:10.1093/ehjci/jer316.
- Yang HS, Bansal RC, Mookadam F, Khandheria BK, Tajik AJ, Chandrasekaran K.: Practical guide for three-dimensional transthoracic echocardiography using a fully sampled matrix array transducer. J Am Soc Echocardiogr. 2008; 21:979-89.
- Lang RM, Bierig M, Devereux RB, Flachskampf Fa, Foster E, Pellikka P, et al.: Recommendations for chamber quantification. Eur J Echocardiogr. 2006;7:79-108.
- Jenkins C, Chan J, Hanekom L, Marwick TH.: Accuracy and feasibility of 3-dimensional echocardiography for measurement of left ventricular parameters. J Am Soc Echocardiogr. 2006; 19:1119-28.
- Muharu D, Badano LP, Piccoli G, Gianfagna P, Del Mestre L, Ermacora D, et al,: Validation of a novel automated border-detection algorithm for rapid and accurate quantification of left ventricular based on three-dimensional echocardiography. Eur J Echocardiogr. 2010;11:359-68.